Alternative Drying Technologies

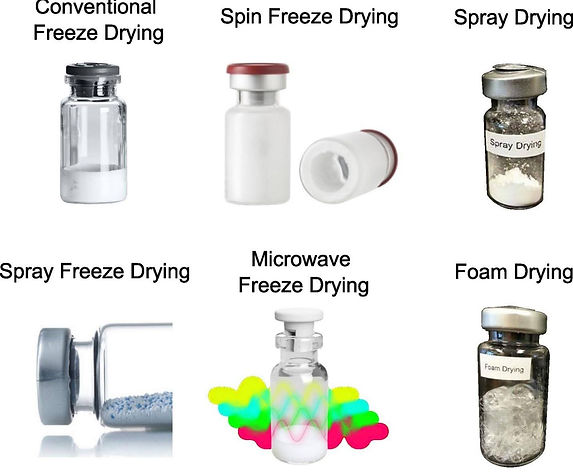
Freeze drying is the most widely used technique for improving the stability of pharmaceutical compounds(1) with the vial freeze-drying (VFD) being the gold standard process for preservation of biologics and temperature sensitive small molecule pharmaceuticals. Despite recent advances in process design(2) and optimization, it remains a long and expensive process. The recent COVID-19 pandemic revealed the lack of lyophilization capacity within industry and exposed the fragility of the pharmaceutical supply chain(3). To overcome these challenges, alternatives to the vial freezedrying process should be explored. There are a few technologies that are available to date at the commercial scale to produce injectable pharmaceuticals: aseptic spray drying(4) (SD), aseptic spray freeze-drying(3) (SFD-for temperature sensitive products) and spin freeze-drying(5) (SpFD). The general tradeoff between these techniques is either a higher throughput (SD and SFD) or greater homogeneity (SpFD) of dry products. While these technologies are available, their application is currently limited for manufacturing of injectable drugs for many technological and logistical reasons(6).
​
Spray drying (SD) is well known drying technique(4,7,8,9) widely used in food industry and pharmaceutical applications. Current advances in liquid atomization and powder-collection technologies have enabled manufacturing of sterile powder with desired characteristics4, with additional benefits of continuous manufacturing. According to A.Siew(4) , SD powders can be engineered to have a good flowability and compactability, enabling an easy filling into various containers including vials, pouches, and medical devices. The drawbacks for SD are sterility assurance and the production of a relatively high moisture of dry powder. The latter precludes the use of SD for moisture sensitive products (<1%), unless additional drying step is included.
Spray freeze-drying (SFD) is a technique that takes advantage of characteristics of spray drying, which involves the atomization of a liquid to create smaller particles, and freeze-drying, which has particular value for drying thermally sensitive materials, to create dry powders in controlled size and enhanced stability(3). Easy flowable, non-compressible powder (pellets) of very low residual moisture (<0.2%) can be aseptically produced using the existing SFD(6) process with the yield above 97%. Excellent flowability allows pellet filling in different types of containers, similar to SD, improving patients/customers convenience. The technology is ready for commercial implementation but the combined efforts of several pharmaceutical companies or contract manufacturing organizations (CMOs) may be needed to share initial capital cost and risk.
​
Spin freeze-drying (SpFD), as a part of a continuous freeze-drying technology, is associated with a much higher drying rate and a higher level of process control in comparison with batch vial freezedrying(10). The temperature and product residual moisture of every individual vial is accurately measured and controlled during short continuous process. The freezing process within spinning vial could be easily and reproducibly controlled in a wide range of cooling and freezing rates not available in large scale VFD. While this process cannot yet compete with VFD in regard to throughput, it can be successfully applied to manufacture of high value/low volume products (RNA based pharmaceuticals(11,12), gene therapy products etc.). The technology is also ready for commercial implementation pending demonstration of a GMP clinical/commercial scale unit.
​
Microwave assisted freeze-drying (MWFD) relies on electromagnetic energy to heat the target material. This technique has been widely used for the preservation of food since the 1960’s and is now being investigated by the pharmaceutical freeze-drying community(1). Several materials including proteins(13) and vaccines(14) have already been tested and exhibit comparable efficacy to control materials dried using conventional freeze-drying. The key advantage of MWFD is the ability to volumetrically heat materials within the product chamber. Conventional freeze-drying technology relies on conduction between the shelf and primary packaging to offset the latent heat of sublimation. Although simple, this technique introduces significant thermal gradients into the frozen materials, limits the usable chamber volume, and is slow to respond to changes in setpoint. In MWFD, a properly mixed high-frequency electric field provides uniform heat input throughout the entire product chamber with high efficiency. This capability provides significant value to both stateof-the-art and emerging drying technologies. For example, one of drawback of otherwise efficient SFD process is some heterogeneity in heat transfer during drying due to a reliance on conduction and infrared heat transfer. Recent advances in microwave technology (RF assisted SFD(13)) could significantly improve the process by accelerating drying rates, increasing throughput (i.e., the amount of material processed per batch), and improving heating uniformity. A similar value proposition has also been proposed for VFD, especially for nested systems (e.g., ready-to-use containers and syringes). Active areas of research for MWFD currently under investigation include heating uniformity, dielectric characterization, and developing robust product temperature measurement technologies.
​
In the next decade, electrostatic spray drying(16) could play more significant role in preservation of biologics and small molecule pharmaceuticals adding to portfolio of preservation technologies that could complement or even replace existing vial freeze-drying process. A number of other drying technologies1 (convective air/nitrogen drying, vacuum drying, foam drying, supercritical fluid drying, acoustic assisted drying etc.) could potentially emerge as viable methods for the aseptic manufacturing of pharmaceuticals, including injectables.
​
References:
-
R. Walters, B. Bhatnagar, S. Tchessalov, K-I. Izutsu, K. Tsumoto, and S. Ohtake, Next-generation drying technologies for pharmaceutical applications. Journal of Pharmaceutical Sciences (2014) Sep; 103 (9):2673-95.
-
Tchessalov, S., Maglio, V., Kazarin, P., Alexeenko, A., Bhatnagar, B., Sahni, E, Shalev, E. Practical Advice on Scientific Design of Freeze-Drying Process: 2023 Update. Pharm Res (2023). https://doi.org/10.1007/s11095-023-03607-9
-
M.B. Adali , A. A. Barresi , G. Boccardo, R. Pisano. Spray Freeze-Drying as a Solution to Continuous Manufacturing of Pharmaceutical Products in Bulk, Processes 2020, 8, 709; doi:10.3390/pr8060709
-
A.Siew. Exploring the use of aseptic spray drying in the manufacture of biopharmaceutical injectables: aseptic spray drying provides an alternative to lyophilization as an enabling stabilization technology for parenteral biologic formulations, Pharmaceutical Technology, Vol. 40, Issue 7, 2016
-
L. De Meyer, P.-J. Van Bockstal, J. Corver, C. Vervaet, J.P. Remon, T. De Beer. Evaluation of spin freezing versus conventional freezing as part of a continuous pharmaceutical freeze-drying concept for unit doses, Int. J. of Pharmaceutics, Volume 496, Issue 1, 30 December 2015, Pages 75-85
-
S.Tchessalov, Spray Freeze-Drying: Road to Commercial Implementation, NIPTE freeze-drying conference, Breckenridge, August 1-4, 2023
-
Vehring, R. Pharmaceutical particle engineering via spray drying. Pharmaceutical Research 2007, 25, 999–1022.
-
A.Langford, S.Ohtake. Spray Drying of Biotherapeutic Compounds, Parenteral Medications, CRC Press, 4th edition, publisher Taylor & Francis, 2019
-
M.Bowen, R. Turok, Yuh-Fun Maa. Spray Drying of Monoclonal Antibodies: Investigating Powder-Based Biologic Drug Substance Bulk Storage, Drying Technology, 31: 1441–1450, 2013
-
J. Lammens, N.M. Goudarzi, L.Leys, G.Nuytten, Pieter-Jan Van Bockstal, C.Vervaet ,M. N. Boone,
-
De Beer 4. Spin Freezing and Its Impact on Pore Size, Tortuosity and Solid State,
-
Pharmaceutics 2021, 13(12), 2126; https://doi.org/10.3390/pharmaceutics13122126
-
S. Meulewaeter, G. Nuytten, M.H.Y. Cheng, S.C. DeSmedt, P.R. Cullis, T. De Beer, I. Lentacker, R. Verbeke. Continuous freeze-drying of messenger RNA lipid nanoparticles enables storage at higher temperatures, Journal of Controlled Release, V.357, May 2023, Pages 149-160
-
P. Merckx, J. Lammens, G. Nuytten, B. Bogaert, R. Guagliardo, T. Maes, C. Vervaet, T. De Beer, S. C. De Smedt, K. Raemdonck. Lyophilization and nebulization of pulmonary surfactant-coated nanogels for siRNA inhalation therapy, European Journal of Pharmaceutics and Biopharmaceutics, V. 157, Dec.2020, Pages 191-199
-
J. H. Gitter, R. Geidobler, I. Presser, G.Winter. Significant Drying Time Reduction Using Microwave-Assisted Freeze-Drying for a Monoclonal Antibody. Journal of Pharmaceutical Sciences, Rapid Communication, V.107, Oct.2018, Issue 10, p.2538-2543.
-
A. Bhambhani, J. Stanbro, D. Roth, E. Sullivan, M. Jones, R. Evans, J. Blue. Evaluation of Microwave Vacuum Drying as an Alternative to Freeze-Drying of Biologics and Vaccines: the Power of Simple Modeling to Identify a Mechanism for Faster Drying Times Achieved with Microwave, AAPS PharmSciTech 22, 52 (2021). https://doi.org/10.1208/s12249-020-01912-9
-
T.T. Mutukuri, A. Darwish, A. Strongrich, D.Peroulis, A.Alexeenko, Q.Zhou, (2022). Radio Frequency - Assisted Ultrasonic Spray Freeze Drying for Pharmaceutical Protein Solids. Journal of Pharmaceutical Sciences. 112. 10.1016/j.xphs.2022.09.024.
-
T. T. Mutukuri, Yuh-Fun Maa, B. Gikanga, R. Sakhnovsky, Q. T. Zhou. Electrostatic spray drying for monoclonal antibody formulation. International Journal of Pharmaceutics, Volume 607, 25 September 2021, 120942
-
R. Walters, B. Bhatnagar, S. Tchessalov, K-I. Izutsu, K. Tsumoto, and S. Ohtake, Next-generation drying technologies for pharmaceutical applications. Journal of Pharmaceutical Sciences (2014) Sep; 103 (9):2673-95.
-
Tchessalov, S., Maglio, V., Kazarin, P., Alexeenko, A., Bhatnagar, B., Sahni, E, Shalev, E. Practical Advice on Scientific Design of Freeze-Drying Process: 2023 Update. Pharm Res
-
M.B. Adali , A. A. Barresi , G. Boccardo, R. Pisano. Spray Freeze-Drying as a Solution to Continuous Manufacturing of Pharmaceutical Products in Bulk, Processes 2020, 8, 709; doi:10.3390/pr8060709
-
A.Siew. Exploring the use of aseptic spray drying in the manufacture of biopharmaceutical injectables: aseptic spray drying provides an alternative to lyophilization as an enabling stabilization technology for parenteral biologic formulations, Pharmaceutical Technology, Vol. 40, Issue 7, 2016
-
L. De Meyer, P.-J. Van Bockstal, J. Corver, C. Vervaet, J.P. Remon, T. De Beer. Evaluation of spin freezing versus conventional freezing as part of a continuous pharmaceutical freeze-drying concept for unit doses, Int. J. of Pharmaceutics, Volume 496, Issue 1, 30 December 2015, Pages 75-85
-
S.Tchessalov, Spray Freeze-Drying: Road to Commercial Implementation, NIPTE freeze-drying conference, Breckenridge, August 1-4, 2023
-
Vehring, R. Pharmaceutical particle engineering via spray drying. Pharmaceutical Research 2007, 25, 999–1022.
-
A.Langford, S.Ohtake. Spray Drying of Biotherapeutic Compounds, Parenteral Medications, CRC Press, 4th edition, publisher Taylor & Francis, 2019
-
M.Bowen, R. Turok, Yuh-Fun Maa. Spray Drying of Monoclonal Antibodies: Investigating Powder-Based Biologic Drug Substance Bulk Storage, Drying Technology, 31: 1441–1450, 2013
-
J. Lammens, N.M. Goudarzi, L.Leys, G.Nuytten, Pieter-Jan Van Bockstal, C.Vervaet ,M. N. Boone,
-
De Beer 4. Spin Freezing and Its Impact on Pore Size, Tortuosity and Solid State,
-
Pharmaceutics 2021, 13(12), 2126; https://doi.org/10.3390/pharmaceutics13122126
-
S. Meulewaeter, G. Nuytten, M.H.Y. Cheng, S.C. DeSmedt, P.R. Cullis, T. De Beer, I. Lentacker, R. Verbeke. Continuous freeze-drying of messenger RNA lipid nanoparticles enables storage at higher temperatures, Journal of Controlled Release, V.357, May 2023, Pages 149-160
-
P. Merckx, J. Lammens, G. Nuytten, B. Bogaert, R. Guagliardo, T. Maes, C. Vervaet, T. De Beer, S. C. De Smedt, K. Raemdonck. Lyophilization and nebulization of pulmonary surfactant-coated nanogels for siRNA inhalation therapy, European Journal of Pharmaceutics and Biopharmaceutics, V. 157, Dec.2020, Pages 191-199
-
J. H. Gitter, R. Geidobler, I. Presser, G.Winter. Significant Drying Time Reduction Using Microwave-Assisted Freeze-Drying for a Monoclonal Antibody. Journal of Pharmaceutical Sciences, Rapid Communication, V.107, Oct.2018, Issue 10, p.2538-2543.
-
A. Bhambhani, J. Stanbro, D. Roth, E. Sullivan, M. Jones, R. Evans, J. Blue. Evaluation of Microwave Vacuum Drying as an Alternative to Freeze-Drying of Biologics and Vaccines: the
-
Power of Simple Modeling to Identify a Mechanism for Faster Drying Times Achieved with Microwave, AAPS PharmSciTech 22, 52 (2021). https://doi.org/10.1208/s12249-020-01912-9
-
T.T. Mutukuri, A. Darwish, A. Strongrich, D.Peroulis, A.Alexeenko, Q.Zhou, (2022). Radio Frequency - Assisted Ultrasonic Spray Freeze Drying for Pharmaceutical Protein Solids. Journal of Pharmaceutical Sciences. 112. 10.1016/j.xphs.2022.09.024.
-
T. T. Mutukuri, Yuh-Fun Maa, B. Gikanga, R. Sakhnovsky, Q. T. Zhou. Electrostatic spray drying for monoclonal antibody formulation. International Journal of Pharmaceutics, Volume 607, 25 September 2021, 120942
